Stem Cells in Regenerative Medicine
Evidence-based therapies for repair of neurologic & orthopedic conditions
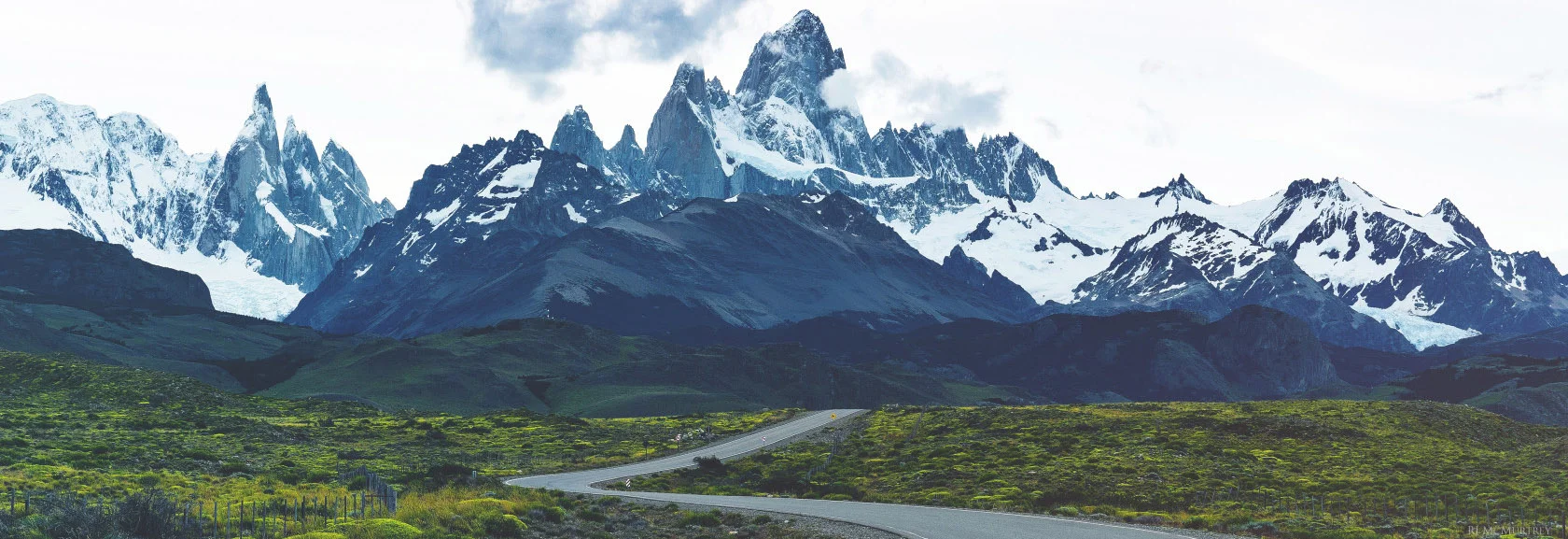
Many new regenerative medicine techniques and technologies are being developed which can help stimulate repair and regeneration of injured or degenerated tissues, and we keep up to date with the latest evidence-based research and clinical studies to provide you with the latest ground-breaking interventions. Regenerative medicine utilizes several approaches for reconstructing damaged tissue and treating painful or degenerative conditions, including the use of specific types of stem cells, growth factors, signaling molecules, peptides, plasma, platelets, exosomes, biomaterials, hydrogels, and/or engineered 3D matrix constructs, along with minimally-invasive image-guided placement of these products directly at the site of injury or degeneration.
"Orthopedic surgeons are at an exciting crossroads in medicine, where biologic therapies are evolving and increasingly available. Time-tested interventions such as arthroplasty have shown good results and still have a major role to play, but newer regenerative approaches have the potential to effectively delay or reduce the requirement for such invasive procedures." --Aaron Krych and Mario Hevesi, Orthopedic Surgeons at the Mayo Clinic, International Orthopaedics, Feb 2021 [1].
"Orthobiologics" is a term for regenerative agents that can accelerate healing and recovery from injuries and can help avoid surgery, instead healing the root cause of the problem more naturally and more completely. For example, mesenchymal stem cells (MSCs) are multipotent cells that can be harvested from your own body and differentiate into a variety of cell types including osteoblasts (for bone), chondrocytes (for cartilage), tenocytes (for tendon), myocytes (for muscle and heart), endothelium and smooth muscle cells (for blood vessels and vascular perfusion), and possibly even neuroglial cell types (for neural tissue) [74-90, 154-163]. We use highly specialized protocols to painlessly harvest, purify, and concentrate stem cells, exosomes, peptides, and growth factors from bone marrow aspirate for your particular needs. We optimize stem cell grafts by sticking them onto the injured tissue under image-guidance, which helps the cellular grafts integrate and rebuild new tissue both by cell receptors and biomechanical cues that adapt cells to the appropriate lineage. By using your own mesenchymal stem cells you use fresh healthy cells that are primed to rebuild your own tissue without immune rejection and thereby avoid stem cell rejection and immune reactions to foreign donor cells.
We are the first institute to combine stem cells with platelet-rich fibrin (PRF) to synergistically increase stem cell survival, integration, and release of growth factors at a wide variety of target sites with an array of complex image-guided injections. When injected into joints and cartilage defects, MSCs with PRF can help patch or pave these injuries for enhanced healing (like re-paving a pothole in the road) and thereby regenerate new hyaline cartilaginous matrix even in full-thickness articular cartilage defects [2-10, 24-50, 131a-b], and this combination of Stem Cells + PRF is also being studied in injuries of tendon, ligament, labrum, meniscus, disc, nerve, and many other tissues [53-73]. Without an adherence matrix like PRF, the stem cells are not fully enabled to properly bind and integrate into these orthopedics tissues and instead tend to undergo a programmed cell death called apoptosis and anoikis.
We are the premier clinic with both the surgical expertise and the stem cell expertise to optimally combine stem cells with your own purified PRF under proper conditions to maximize their survival, viability, and repair capacity with minimal processing, then immediately inject these fresh cells directly at injury sites under image-guidance for maximizing their tissue repair properties and proper 3D tissue reconstruction based on your specific injuries. PRF acts as a biologic glue and anchor to hold the stem cells at the injury site and aid their integration. Dr. McMurtrey spent several years researching stem cell cultures and applications at some of the top university labs in the world, including at the University of Oxford where his thesis on stem cells, bioregenerative tissue engineering, and 3D tissue reconstruction earned the highest honor of distinction and resulted in several publications in top-ranked prestigious research journals.
PRF can capture your own growth factors and small numbers of circulating bone-marrow stem cells (including hematopoietic and/or mesenchymal) [11-16] and PRF uses your own cells and activated platelets to focally release growth factors such as BMP, FGF, VEGF, PDGF, eNOS, and others which are released locally over the next few days to weeks. These factors can help accelerate and strengthen tissue repair, and these further activate recruitment and differentiation of MSCs as well as expression of several tissue remodeling genes [3-7]. MSCs may also have many other good anti-inflammatory, immunomodulatory, reparative, and/or vascularization effects. These stem cells not only can help rebuild damaged tissues, but also secrete growth factors and additional signaling cues in packets known as exosomes, which can further suppress deleterious inflammatory/degenerative pathways to help kickstart cells out of a degenerative rut and back into a high-productivity regenerative phase that favors tissue healing, cellular repair, and strengthening of damaged structures. Thus in addition to powerful cell-based therapies, exosomes and peptides are also being studied as a cell-free solution for injection into or around sites of injured tissues and organs [91-102]. All these options can be administered in our clinic using minimally-invasive injections under high-resolution image-guidance for optimal repair of numerous types of injuries.
CLINICAL EVIDENCE FOR STEM CELLS:
There are several clinical studies in progress on the benefits of stem cell injections for many ailments like arthritic and damaged joints, tendon tears and connective tissue injuries, spine and spinal cord injuries, peripheral nerve injuries, stroke, brain injuries, neurodegenerative diseases, and many other forms of organ and tissue damage. For example, studies have already shown benefits and safety in knee injuries and knee osteoarthritis [37-40, 65-73]. Further clinical studies are still ongoing for several spine, nerve, brain, spinal cord, central nervous system, and neurological conditions like stroke, traumatic injury, and neurodegeneration [42-43, 51, 74-90, 95-97, 134, 154, 162-163], and autologous bone marrow stem cell transplants have also demonstrated some of the best effects on controlling auto-immune conditions including rheumatoid arthritis, reactive arthritis, lymee arthritis, scleroderma, Crohn's disease, and neuro-immunological diseases like multiple sclerosis by rebooting the immune system through a re-diversification of naive and regulatory immune effector cells and other immunosuppressive effects [139-142]. Thus stem cells not only integrate and rebuild tissues directly for long-lasting repair, but also release growth factors, exosomes, and other factors that trigger long-lasting adaptive tissue repair responses.
Stem cells have also shown significant benefits in neurodegenerative diseases such as amyotrophic lateral sclerosis (ALS), which is characterized by degeneration of upper and lower motor neurons, with mesenchymal stem cells possibly delaying progression of this disease and even giving partial recovery of neuron function [112-116]. Bone marrow stem cells appear to be especially effective in ALS, with one clinical study showing that 87% of stem-cell treated ALS patients had at least 25% improvement in function within 6 months of autologous bone-marrow-derived mesenchymal stem cell treatment [113]. These benefits are thought to arise at least in part due to the neurotrophic factors, growth factors, and other signaling cues that are produced and excreted by the stem cells to accelerate tissue repair [113-114, 117]. Stem cell factors such as VEGF, angiogenin, and TGF-β seem to be higher in those patients who have the best response to the stem cell therapy, and these stem cells can be delivered by epidural, intrathecal, intravenous, intranasal, intramuscular, and much more specific image-guided injections [113-114]. Mesenchymal stem cells have also been used to form a "stem cell patch" to repair degenerative disc defects, annular tears, and spina bifida defects around the spinal cord to prevent paralysis in infants and possibly even reverse and repair damage to spinal cord and nerve function [51, 79, 84-86, 89-90, 95-96, 135-145, 198-200]. One of the mechanisms of stem cell healing for herniated spinal discs and nerve compressions may be not only direct repair of the injured tissues and strengthening of the disc, but also proper suppression of the painful inflammatory immune signaling around pathological sites [137].
Bone marrow stem cells have also been used to successfully treat traumatic spine injuries, traumatic brain injuries and concussions by strengthening spinal tissue repair and preserving critical brain architecture as well as suppressing inflammatory signaling [42-43, 74-90, 97, 134-138, 162], and this effect may be augmented by temporary non-invasive blood-brain barrier opening using ultrasonic trans-cranial doppler while infusing the cells directly in the clinic. In addition, the body’s own stores of fat tissue, called subcutaneous adipose tissue as well as stromal-vascular fractions (SAT and SVF), also contain multipotent stem cells of the Schwann cell class that can differentiate into both neurons and glial cells, making yet another potential source of powerful cells for potentially treating a variety of neurological injuries and conditions [118-119]. We are particularly interested in using the power of your own purified bone marrow stem cells to stimulate BDNF release, promote remodeling of neural networks and gliosis, and inhibit neuroinflammation [113, 163-167] for applications in spinal cord injuries and traumatic brain injuries.
Furthermore, a recent study showed that many commercial stem cell products, including those derived from wharton's jelly, umbilical, amniotic, or other perinatal sources, actually contained very few (if any) viable stem cells at all since most die in the storage and processing [36]. This study then compared fresh stem cells from a patients' own bone marrow mesenchymal stem cells and showed that these stem cells were abundant even in elderly patients and retained dramatic abilities to live, grow, proliferate, and differentiate into tissue-specific subtypes [36], plus these cells can build new tissue without causing an immune reaction (unlike foreign donor cells). Bone marrow stem cells are already primed for repair of many types of mesenchymal tissues, thus your own body is the best source for stem cell harvesting and injury repair in most cases, especially orthopedic-type tissue injuries, but these cells need to be placed into the proper location with proper conditions to best repair tissues. If there is any doubt about the power of stem cells, remember that well into late age the body retains stem cells that can create entirely new offspring with perfect forms of every tissue structure-- we just need to harness the potential of our own stem cells to optimize and steer their incredible capabilities. Interestingly, animals like salamanders and axolotl are able to regenerate entire limbs, and this regenerative process is driven primarily by hematopoietic and myeloid stem cells, which in the human is the equivalent of bone marrow stem cells that drive mesenchymal cell lineages of ligament, cartilage, muscle, bone, and connective tissues [52].
Depending on your needs, we can harvest stem cells from your own bone marrow with a simple needle and numbing sedation, and we use specialized surgical protocols to maximize isolation, yield, viability, and long-term survival of the stem cells with minimal processing and immediate injection of the fresh cells back into your body in the same procedure. These types of autologous bone grafts and bone marrow procedures have been done in many types of surgical procedures for many different reasons. Research has elucidated many ways that these stem cells are primed for tissue repair and can be used to help stimulate your own natural healing, reconstruction, or repair of injured or degenerated tissues and joints such as muscle, ligaments, tendons, nerves, bones, cartilage, and fibrocartilage [2-10, 17-51, 83-90, 120-132]. Interestingly, organs like the heart and brain may also benefit from stem cell treatments, as there are also other hormones and peptides in bone marrow like osteocalcin which have been shown to enhance memory, brain function, cardiac function, fitness, metabolism, healing, and tissue remodeling after ischemic damage [120-132, 151-161]. Bone marrow stem cells have also shown promise in repairing and healing many orthopedic injuries including non-union fractures, failed surgeries, pseudoarthrosis, spondylolysis, pars defects, joint injuries, arthritis, cartilage damage and defects, as well as many other complex conditions [124, 146-153, 194-197]. These bone-marrow cells can be injected at any area in the body under image-guidance, and they also have good evidence for helping repair and rebuild osteonecrosis, avascular necrosis, bone marrow failure, spine and disc injuries, and subchondral defects in bones and joints [168-178, 194-197], which provides immense hope for patients with these recalcitrant and refractory conditions. The addition of certain peptides with stem cells may further enhance bone reunionization and healing of bone fractures, ligament tears, and tendon attachment avulsions [178-193]. Other common sites for bone marrow stem cell injections include spine, discs, nerves, knees, hips, shoulders, ankles, feet, sacroiliac joint, elbows, hands, covering a wide variety of traumatic injuries and tissue defects, avulsions, fractures, tears, neuropathies, and other chronic non-healing injuries. All these procedures are best done under live image-guidance to ensure accurate and specific targeting of the injury site with appropriate localization of cells and scaffolding with growth factors for optimal regeneration and healing.
OVERVIEW OF SOME STEM CELL SIGNALING PATHWAYS:

Finally, the history of stem cell research is also fascinating and worth briefly covering here. The earliest concepts of stem cells arose in the early 1900s when studying the ability of bone marrow to continually produce new blood cells. Then as a graduate student in 1958, John Gurdon discovered in his research work at Oxford that mature cells retain all the information needed to make any cell type of the body encoded somewhere deep within their own DNA [103]. Many years later, a physician named Shinya Yamanaka was pursuing his training in orthopedic surgery when he began to notice how many diseases and conditions exist that physicians actually have no ability to fix or heal (including everything from spinal cord injuries and traumatic brain injuries to orthopedic conditions and autoimmune diseases), and he lost confidence in the surgical approach and instead turned his efforts towards curing diseases from a much more thoughtful scientific approach, which ultimately resulted in one of the greatest discoveries of all time (winning a Nobel Prize in 2012): mature adult cells can in fact be reverted back to a full pluripotent stem cell state, capable of becoming any type of cell or tissue, by just introducing a few simple factors into the cell [104]. Dr. McMurtrey has since replicated this work in his own lab, taking adult blood cells and reprogramming them back into pluripotent stem cells, then differentiating and directing them to reconstruct new 3D tissue types using a variety of biochemical, biomechanical, and nanopatterning cues [105-111].
REFERENCES:
(1) Advancing regenerative surgery in orthopaedic sports medicine:
the critical role of the surgeon
(2) The Clinical Use of Human Culture-Expanded Autologous Bone
Marrow Mesenchymal Stem Cells Transplanted on Platelet-Rich Fibrin
Glue in the Treatment of Articular Cartilage Defects
(3) Platelet-Rich Fibrin and Its Emerging Therapeutic Benefits for
Musculoskeletal Injury Treatment
(4) Platelet-Rich Fibrin Scaffolds for Cartilage and Tendon
Regenerative Medicine: From Bench to Bedside
(5) From Platelet-Rich Plasma to Advanced Platelet-Rich Fibrin:
Biological Achievements and Clinical Advances in Modern Surgery
(6) Leucocyte and Platelet‐rich Fibrin: a carrier of autologous
multipotent cells for regenerative medicine
(7) Cytokine and Growth Factor Delivery from Implanted
Platelet-Rich Fibrin Enhances Rabbit Achilles Tendon Healing
(8) Cartilage Defect Treatments: A Systematic Review and
Meta-Analyses
(9) Regenerative approaches for the treatment of early OA
(10) Stem Cells in Osteochondral Tissue Engineering
(11) Circulating stem cells
(12) Peripheral blood and bone marrow hematopoietic stem cells: are
they the same?
(13) Exercise-Induced Circulating Hematopoietic Stem and Progenitor
Cells in Well-Trained Subjects
(14) Circulating mesenchymal stem cells
(15) Circulating Stem Cells in Physiology and Pathology - Recent
Studies Published in Stem Cell Reviews and Reports
(16) Circulating Bone Marrow-Derived Osteoblast Progenitor Cells
Are Recruited to the Bone-Forming Site by the CXCR4/Stromal
Cell-Derived Factor-1 Pathway
(17) Clinical Trials With Mesenchymal Stem Cells: An Update
(18) Human Adipose-Derived Stem Cells with Great Therapeutic
Potential
(19) Stem cells: their source, potency and use in regenerative
therapies with focus on adipose-derived stem cells - a review
(20) Current Strategies to Enhance Adipose Stem Cell Function: An
Update
(21) Adipose-derived mesenchymal stem cells: biology and potential
applications
(22) Adult Stem Cells for Regenerative Therapy
(23) Multifaceted Characterization of Human Mesenchymal Stem Cells
for Use in Regenerative Medicine
(24) Mesenchymal Stem Cells for Regenerative Medicine
(25) Regenerative Capacity of Adipose Derived Stem Cells (ADSCs),
Comparison with Mesenchymal Stem Cells (MSCs)
(26) Adult mesenchymal stem cells and their exosomes: Sources,
characteristics, and application in regenerative medicine
(27) Mesenchymal Stem/Stromal Cells in Regenerative Medicine: Past,
Present, and Future
(28) Regenerative Cardiovascular Therapies: Stem Cells and
Beyond
(29) Intraarticular injection of bone marrow-derived mesenchymal
stem cells enhances regeneration in knee osteoarthritis
(30) Bone marrow mesenchymal stem cell-derived exosomes protect
cartilage damage and relieve knee osteoarthritis pain in a rat model
of osteoarthritis
(31) Stem cell-based therapeutic strategies for cartilage defects
and osteoarthritis
(32) Stem Cell Treatment for Knee Articular Cartilage Defects and
Osteoarthritis
(33) Cartilage Regeneration in Humans with Adipose Tissue-Derived
Stem Cells and Adipose Stromal Vascular Fraction Cells: Updated
Status
(34) Cell therapy for cartilage defects of the hip
(35) Mesenchymal stem cell therapy in the treatment of hip
osteoarthritis
(36) Colony Forming Potential and Protein Composition of Commercial
Umbilical Cord Allograft Products in Comparison With Autologous
Orthobiologics
(37) Prospective, Single-Blind, Placebo-Controlled Trial of Bone
Marrow Aspirate Concentrate for Knee Osteoarthritis
(38) Quantitative T2 MRI Mapping and 12-Month Follow-up in a
Randomized, Blinded, Placebo Controlled Trial of Bone Marrow
Aspiration and Concentration for Osteoarthritis of the Knees
(39) Subchondral bone or intra-articular injection of bone marrow
concentrate mesenchymal stem cells in bilateral knee
osteoarthritis
(40) Intraarticular injection of bone marrow-derived mesenchymal
stem cells enhances regeneration in knee osteoarthritis
(41) Application of Bone Marrow-Derived Mesenchymal Stem Cells for
Muscle Healing After Contusion Injury
(42) Comparison of the use of adipose-derived and bone
marrow-derived stem cells for peripheral nerve regeneration in vitro
and in vivo
(43) Comparative Study on Bone Marrow-Versus Adipose-Derived Stem
Cells on Regeneration and Re-Innervation of Skeletal Muscle
Injury
(44) Cartilage Repair With Autologous Bone Marrow Mesenchymal Stem
Cell Transplantation
(45) Chondrogenic Differentiation of Bone Marrow-Derived
Mesenchymal Stem Cells: Tips and Tricks
(46) Mixed cell therapy of bone marrow-derived mesenchymal stem
cells and articular cartilage chondrocytes ameliorates
osteoarthritis development
(47) Mesenchymal stem cells: amazing remedies for bone and
cartilage defects
(48) Use of Adult Stem Cells for Cartilage Tissue Engineering:
Current Status and Future Developments
(49) Mesenchymal stem cells for cartilage regeneration
(50) GREM1 suppresses hypertrophy of engineered cartilage in vitro
but not bone formation in vivo
(51) NeuroRegen Scaffolds Combined with Autologous Bone Marrow
Mononuclear Cells for the Repair of Acute Complete Spinal Cord
Injury: A 3-Year Clinical Study
(52) Identification of Hematopoietic Cells as the Primary Reservoir
for Pro-regenerative Macrophages Required for Salamander Limb
Regeneration
(53) The effect of L-PRF membranes on bone healing in rabbit tibiae
bone defects: micro-CT and biomarker results
(54) Positive effects of different plasma methods on human muscle,
bone, and tendon cells
(55) Influence of plasma on chondrogenic differentiation and
proliferation of chondrocytes and mesenchymal stem cells
(56) Proliferation-promoting effect of plasma on human
adipose-derived stem cells and human dermal fibroblasts
(57) Plasma enhances mesenchymal stem cell proliferation and
chondrogenic differentiation
(58) Human plasma stimulates migration and chondrogenic
differentiation of human subchondral progenitor cells
(59) Adult and umbilical cord blood-derived plasma for mesenchymal
stem cell proliferation, chemotaxis, and cryo-preservation
(60) Comparison of surgically repaired Achilles tendon tears using
platelet-rich fibrin matrices
(61) Treatment of tendon and muscle using plasma
(62) Plasma compared with corticosteroid injection for chronic
lateral elbow tendinosis
(63) Effects of repetitive plasma application on human tenocyte
proliferation
(64) Regenerative potentials of plasma enhanced by collagen in
retrieving pro-inflammatory cytokine-inhibited chondrogenesis
(65) Human platelet lysate successfully promotes proliferation and
subsequent chondrogenic differentiation of adipose-derived stem
cells: a comparison with articular chondrocytes
(66) The Role of Intraarticular Platelet Rich Plasma Injection in
Patients with Internal Knee Derangements
(67) Percutaneous injections of Platelet rich plasma for treatment
of intrasubstance meniscal lesions
(68) Intra-articular Platelet-Rich Plasma Injections for Treating
Knee Pain Associated with Articular Cartilage and Degenerative
Meniscal Lesions
(69) Treatment of degenerative meniscal tear with intrameniscal
injection of platelet rich plasma
(70) Ultrasound-Guided Meniscal Injection of Autologous Growth
Factors: A Brief Report
(71) Effects of Platelet-Rich Plasma and Bone Marrow Mesenchymal
Stem Cells on Meniscal Repair in the White-White Zone of the
Meniscus
(72) Efficacy of Autologous Platelet-Rich Plasma Injections for
Grade 3 Symptomatic Degenerative Meniscal Lesions: A 1-Year
Follow-up Prospective Study
(73) Prospective Study Comparing Leukocyte-Poor Platelet-Rich
Plasma Combined with Hyaluronic Acid and Autologous Microfragmented
Adipose Tissue in Patients with Early Knee Osteoarthritis
(74) Bone marrow-derived mesenchymal stem cells differentiate into
nerve-like cells in vitro after transfection with brain-derived
neurotrophic factor gene
(75) Differentiation of Mesenchymal Stem Cells to Neuroglia: in the
Context of Cell Signalling
(76) Effects of Follistatin on the Differentiation of Human Bone
Marrow Mesenchymal Stem Cells into Neurons-Like Cells
(77) In vitro differentiation of human bone marrow stromal cells
into neural precursor cells using small molecules
(78) Interleukin-17A promotes the differentiation of bone marrow
mesenchymal stem cells into neuronal cells
(79) Bone marrow mesenchymal stem cells promote remyelination in
spinal cord by driving oligodendrocyte progenitor cell
differentiation via TNFα/RelB-Hes1 pathway
(80) Neuro-glial differentiation of human bone marrow stem cells in
vitro
(81) Differentiation into neurons of rat bone marrow-derived
mesenchymal stem cells
(82) Neurospheres Induced from Human Adipose-Derived Stem Cells as
a New Source of Neural Progenitor Cells
(83) Comparative study of neural differentiation capacity of
mesenchymal stromal cells from different tissue sources: An approach
for use in neural regeneration therapies
(84) Stem cell therapy for nerve injury
(85) Combining cell therapy with human autologous Schwann cell and
bone marrow-derived mesenchymal stem cell in patients with subacute
complete spinal cord injury
(86) Stem cell therapies for acute spinal cord injury in humans: a
review
(87) Adipose Tissue and Mesenchymal Stem Cells: State of the Art
and Lipogems Technology Development
(88) Mesenchymal stem cell transplantation in amyotrophic lateral
sclerosis: A Phase I clinical trial
(89) A clinical trial report of autologous bone marrow-derived
mesenchymal stem cell transplantation in patients with spinal cord
injury
(90) A Phase III Clinical Trial Showing Limited Efficacy of
Autologous Mesenchymal Stem Cell Therapy for Spinal Cord Injury
(91) Biology and therapeutic potential of mesenchymal stem
cell-derived exosomes
(92) The biology , function , and biomedical applications of
exosomes
(93) MSC-Derived Exosomes for Cell-Free Therapy
(94) Therapeutic application of exosomes in ischaemic stroke
(95) Mesenchymal stem cell-derived exosomes: therapeutic
opportunities and challenges for spinal cord injury
(96) Neural stem cell-derived exosomes facilitate spinal cord
functional recovery after injury by promoting angiogenesis
(97) Exosomes as a Promising Therapeutic Strategy for Peripheral
Nerve Injury
(98) Therapeutic Application of Exosomes in Inflammatory
Diseases
(99) Exosomes: biology, therapeutic potential, and emerging role in
musculoskeletal repair and regeneration
(100) Therapeutic potential of exosomes in rotator cuff tendon
healing
(101) Exosomes in osteoarthritis and cartilage injury: advanced
development and potential therapeutic strategies
(102) Mutant SRF and YAP synthetic modified mRNAs drive
cardiomyocyte nuclear replication
(103) John Gurdon - Nobel Prize Biographical Essay
(104) Shinya Yamanaka - Nobel Prize Biographical Essay
(105) Analytic Models of Oxygen and Nutrient Diffusion, Metabolism
Dynamics, and Architecture Optimization in Three-Dimensional Tissue
Constructs with Applications and Insights in Cerebral Organoids
(106) Patterned and functionalized nanofiber scaffolds in
three-dimensional hydrogel constructs enhance neurite outgrowth and
directional control
(107) Novel advancements in three-dimensional neural tissue
engineering and regenerative medicine
(108) Roles of Diffusion Dynamics in Stem Cell Signaling and
Three-Dimensional Tissue Development
(109) Multi-compartmental biomaterial scaffolds for patterning
neural tissue organoids in models of neurodevelopment and tissue
regeneration
(110) Organoids and Mini-Organs: Elements of organoid design
(111) Co-Electrospun Silk Fibroin and Gelatin Methacryloyl Sheet
Seeded with Mesenchymal Stem Cells for Tendon Regeneration
(112) The Safety and Efficacy of Stem Cell Therapy as an Emerging
Therapy for ALS: A Systematic Review of Controlled Clinical Trials
(113) Safety and Clinical Effects of Mesenchymal Stem Cells
Secreting Neurotrophic Factor Transplantation in Patients With
Amyotrophic Lateral Sclerosis: Results of Phase 1/2 and 2a Clinical
Trials
(114) Amyotrophic lateral sclerosis: promising therapeutic
outcome–not far away?
(115) Mesenchymal Stem Cells: A Potential Therapeutic Approach for
Amyotrophic Lateral Sclerosis?
(116) Stem cell treatments for amyotrophic lateral sclerosis: a
critical overview of early phase trials
(117) Cytoplasm or Supernatant: Where Is the Treasury of the
Bioactive Antiaging Factor from Mesenchymal Stem Cells?
(118) Schwann cells in the subcutaneous adipose tissue have
neurogenic potential and can be used for regenerative therapies
(119) Fat could hold key to stem cell treatments for Parkinson's
disease
(120) Mediation of the Acute Stress Response by the Skeleton
(121) Maternal and offspring pools of osteocalcin influence brain
development and functions
(122) Osteocalcin Signaling in Myofibers Is Necessary and
Sufficient for Optimum Adaptation to Exercise
(123) Using Stem Cell Therapies to Reestablish Osteogenic
Capability for Bone Regeneration
(124) Adult Stem Cells for Bone Regeneration and Repair
(125) Prospect of Stem Cell Therapy and Regenerative Medicine in
Osteoporosis
(126) The Current Perspectives of Stem Cell Therapy in Orthopedic
Surgery
(127) BMP-9 induced osteogenic differentiation of mesenchymal stem
cells: molecular mechanism and therapeutic potential
(128) Clinical evaluation following the percutaneous
transplantation of allogenic bone marrow-derived mesenchymal stem
cells (aBM-MSC) in dogs affected by vertebral compression
fracture
(129) Mesenchymal Stem Cells for Bone Repair and Metabolic Bone
Diseases
(130) Systemic Adult Stem Cell Therapy for Osteoporosis-Related
Vertebral Compression Fractures
(131a) Mesenchymal stem cells: amazing remedies for bone and
cartilage defects
(131b) Peptide Regulation of Chondrogenic Stem Cell Differentiation
(132a) Lipid availability determines skeletal progenitor cell fate
via SOX9
(132b) Mesenchymal stem cell implantation provides short-term clinical improvement and satisfactory cartilage restoration in patients with knee osteoarthritis but the evidence is limited: a systematic review
(133) A new neutrophil subset promotes CNS neuron survival and axon
regeneration
(134) CIRM Clinical Trials
(135) World-first stem cell therapy trial treats spina bifida
before birth
(136) Cellular Therapy for In Utero Repair of Myelomeningocele -
The CuRe Trial (CuRe)
(137) Bone mesenchymal stem cells attenuate radicular pain by
inhibiting microglial activation in a rat noncompressive disk
herniation model
(138) Treatment of Severe Adult Traumatic Brain Injury Using Bone
Marrow Mononuclear Cells
(139a) Autologous haematopoietic stem cell transplantation (aHSCT)
for severe resistant autoimmune and inflammatory diseases
(139b) The Life and Fate of Mesenchymal Stem Cells
(140) Hematopoietic stem cell transplantation and cellular
therapies for autoimmune diseases: overview and future
considerations
(141) Stem cell therapy can safely slow progression of
relapsing-remitting MS
(142) Stem cell transplantation for treatment of
relapsing-remitting multiple sclerosis in Sweden: an observational
cohort study
(143) Bone marrow mesenchymal stem cells and exercise restore motor
function following spinal cord injury by activating PI3K/AKT/mTOR
pathway
(144) Mesenchymal Stem Cell Transplantation: Neuroprotection and
Nerve Regeneration After Spinal Cord Injury
(145) Bone marrow mesenchymal stem cells (BMSCs) improved
functional recovery of spinal cord injury partly by promoting axonal
regeneration
(146) Stem Cell Therapy in the Management of Fracture Non-Union –
Evaluating Cellular Mechanisms and Clinical Progress.
(147) The role of stem cells in fracture healing and nonunion.
(148) Percutaneous injection of bone marrow mesenchymal stem cells
for ankle non-unions.
(149) Treatment of Peri-Implant Defects in the Rabbit's Tibia with Adipose or Bone Marrow-Derived Mesenchymal Stems Cells.
(150) Stem cell therapy for fracture non-union: The current
evidence from human studies.
(151) A systematic review of the clinical applications and
complications of bone marrow aspirate concentrate in management of
bone defects and nonunions.
(152) Application of bone marrow mesenchymal stem cells to the
treatment of osteonecrosis of the femoral head.
(153) Cotransplantation of mesenchymal stem cells and endothelial
progenitor cells for treating steroid-induced osteonecrosis of the
femoral head.
(154) Effects of intravenous administration of allogenic bone
marrow- and adipose tissue-derived mesenchymal stem cells on
functional recovery and brain repair markers in experimental
ischemic stroke
(155) Bone marrow-derived cells contribute to infarct
remodelling.
(156) Randomized Trial of Targeted Transendocardial Mesenchymal
Precursor Cell Therapy in Patients With Heart Failure.
(157) Bone Marrow Mesenchymal Stem Cells for Heart Failure
Treatment: A Systematic Review and Meta-Analysis.
(158) The therapeutic potential of mesenchymal stem cells for
cardiovascular diseases.
(159) Mesenchymal stem cell mediates cardiac repair through
autocrine, paracrine and endocrine axes.
(160) Mesenchymal Stem Cells in Cardiology.
(161) Mesenchymal stem cells and cardiac repair.
(162) Mesenchymal stem cells and cardiac repair.
(163) Clinical Trials of Stem Cell Therapy for Cerebral Ischemic
Stroke.
(164) Functional Mechanism of Bone Marrow-Derived Mesenchymal Stem Cells in the Treatment of Animal Models with Alzheimer's Disease: Inhibition of Neuroinflammation.
(165) The functional mechanism of bone marrow-derived mesenchymal stem cells in the treatment of animal models with Alzheimer's disease: crosstalk between autophagy and apoptosis.
(166) Bone marrow-derived mesenchymal stem cells improve cognitive impairment in an Alzheimer's disease model by increasing the expression of microRNA-146a in hippocampus.
(167) Exosomes derived from bone-marrow mesenchymal stem cells alleviate cognitive decline in AD-like mice by improving BDNF-related neuropathology.
(168) Analysis of Results After Surgical Application of Bone Marrow Aspirate Stem Cell Concentrate in the Treatment of Avascular Necrosis of the Femoral Head.
(169) Multiscale Stem Cell Technologies for Osteonecrosis of the Femoral Head.
(170) Autologous bone marrow derived mesenchymal stem cell therapy for osteonecrosis of femoral head: A systematic overview of overlapping meta-analyses.
(171) Mesenchymal stem cells for subchondral bone marrow lesions: From bench to bedside.
(172 Autologous Marrow Mesenchymal Stem Cell Driving Bone Regeneration in a Rabbit Model of Femoral Head Osteonecrosis.
(173) Osteonecrosis repair with bone marrow cell therapies: state of the clinical art .
(174) Stem cell therapy for osteonecrosis of femoral head: Opportunities and challenges .
(175) Stem cell treatment for avascular necrosis of the femoral head: current perspectives.
(176) Autologous stem cell implantation with core decompression for avascular necrosis of the femoral head .
(177) Autologous stem cell implantation with core decompression for avascular necrosis of the femoral head using a new device .
(178) Osteogenic effect of a gastric pentadecapeptide BPC-157 on the healing of segmental bone defect in rabbits: a comparison with bone marrow and autologous cortical bone implantation .
(179) Gastric pentadecapeptide body protection compound BPC 157 and its role in accelerating musculoskeletal soft tissue healing .
(180) The promoting effect of pentadecapeptide BPC 157 on tendon healing involves tendon outgrowth, cell survival, and cell migration .
(181) Effective therapy of transected quadriceps muscle in rat: Gastric pentadecapeptide BPC 157 .
(182) Gastric pentadecapeptide BPC 157 as an effective therapy for muscle crush injury in the rat .
(183) Modulatory effect of gastric pentadecapeptide BPC 157 on angiogenesis in muscle and tendon healing .
(184) Modulation of early functional recovery of Achilles tendon to bone unit after transection by BPC 157 and methylprednisolone .
(185) Achilles detachment in rat and stable gastric pentadecapeptide BPC 157: Promoted tendon-to-bone healing and opposed corticosteroid aggravation .
(186) Gastric pentadecapeptide BPC 157 accelerates healing of transected rat Achilles tendon and in vitro stimulates tendocytes growth .
(187) Pentadecapeptide BPC 157 enhances the growth hormone receptor expression in tendon fibroblasts .
(188) Pentadecapeptide BPC 157 (PL 14736) improves ligament healing in the rat .
(189) BPC 157's effect on healing .
(190) Bioactive peptides for boosting stem cell culture platform: Methods and applications .
(191) Cell-binding peptides on the material surface guide stem cell fate of adhesion, proliferation and differentiation .
(192) The spatial patterning of RGD and BMP-2 mimetic peptides at the subcellular scale modulates human mesenchymal stem cells osteogenesis .
(193) Peptides for bone tissue engineering .
(194) Ultrasound-guided intra-articular injection of expanded umbilical cord mesenchymal stem cells in knee osteoarthritis: a safety/efficacy study with MRI data .
(195) Human bone marrow mesenchymal stem cell injection in subchondral lesions of knee osteoarthritis: a prospective randomized study versus contralateral arthroplasty at a mean fifteen year follow-up .
(196) Role of Scaffolds, Subchondral, Intra-Articular Injections of Fresh Autologous Bone Marrow Concentrate Regenerative Cells in Treating Human Knee Cartilage Lesions .
(197) Subchondral stem cell therapy versus contralateral total knee arthroplasty for osteoarthritis following secondary osteonecrosis of the knee .
(198) Cytokine polarized alternatively activated bone marrow neutrophils drive axon regeneration .
(199) Culturing neural and bone mesenchymal stem cells in photosensitive hydrogel enhances spinal cord injury repair .
(200) Stem cells use “first aid kit" vesicles to repair damage .
-----
ASOI © 2021 All Rights Reserved*Disclaimer: We seek to always provide the highest-quality evidence-based care to our patients customized for their specific conditions, injuries, and diagnoses, which may include FDA-approved therapies as well as additional investigational, alternative, or regenerative therapies. We always discuss potential risks and benefits of all these options. The information presented here is for informational use and cites the ongoing cutting-edge research and medical advancements on these relevant topics. There are many treatments, interventions, and protocols routinely practiced in medicine and surgery which the FDA has not studied nor formally approved yet which have demonstrated overwhelming evidence of efficacy and clinical benefit, while many standard treatments and common surgeries can actually have high rates of failure and complication. The FDA does not regulate the practice of medicine but rather regulates medical marketing of devices and drugs. The FDA does not conduct clinical trials or attempt to discover new treatments, but rather requires companies or other entities to fund marketing approvals. Breakthrough technologies typically require years to decades of research work to optimize the technology and collect enough data to prove efficacy and superiority, which in some cases can optionally be submitted to the FDA if there is sufficient financial backing to market a specific product or drug. Thus the FDA has not yet studied, evaluated, or formally approved many regenerative therapies currently practiced by many of the top physicians and surgeons in the United States and around the world. Some therapies, products, or interventions may still be considered investigational or "off-label" even with substantial evidence of efficacy, and many different applications of regenerative therapies continue to be researched by our institute and other top institutions around the world. The rapid evolution and advancement of medicine demands that physicians continually update their knowledge and practice techniques to adapt to future improvements and advancing technologies. These statements have not been evaluated by the FDA, and the treatments and products presented here are for informational purposes and not guaranteed to diagnose, treat, cure, or prevent any specific disease or condition. All injuries and conditions should be formally evaluated by a knowledgeable medical professional whereby standard treatments and additional therapeutic interventions may be considered with the diagnosis and treatment plan.